The only problem was that her head was on the edge of the field of view. Even though she wasn’t moving, we were capturing only the front half of her brain. This was a direct consequence of setting the field of view without a localizer image. We’d shot blind and missed by an inch.
I let the functional sequence run for the full five minutes. Even if we wouldn’t be able to use her data this time, it was good training for Melissa and McKenzie. When it was done, I gave them the report.
“The good news is that McKenzie held her head still,” I said. “The bad news is that we got only half her brain.”
“McKenzie’s not too tired,” Melissa said. “We could try again.”
“If you could get her to scoot her head forward an inch,” I said, “that would help.”
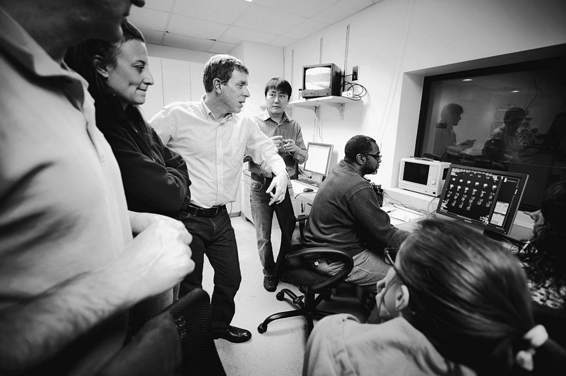
Mark, Melissa, I, Sinyeob, Robert, Lisa, and Kristina study the first functional images.
(Bryan Meltz)
Once again, everyone took their positions, and with McKenzie resettled in the magnet, we went through the protocol for what seemed like the hundredth time that day. This time, her head was closer to the center of the field of view. Some images were still clipped, but overall, the run looked very good.
Between Callie and McKenzie, we had exceeded our goal of acquiring a sequence of ten functional images. Even if we had only a partial scan of McKenzie, we had almost one hundred images of both dogs—enough to do a crude analysis of brain activation comparing hand signals for peas and hot dogs.
When we got home, Callie ran right to the kitchen. Even though she had spent the entire afternoon consuming peas and hot dogs, her appetite had not diminished in the slightest. She stood expectantly next to her food bowl. So, where’s my dinner?
Lyra detected the foreign smells of the hospital and sniffed Callie from head to tail. Satisfied that it was still Callie, Lyra wagged her tail and let out a few yippy barks of recognition.
Helen plopped down on the sofa.
“So,” I said, “what did you think?”
“It was pretty cool.”
“Science doesn’t always go the way they teach it at school,” I said. “You never know what will happen.” I paused and continued. “I’m really glad you came today. It was fun having you there.”
Helen nodded her head, and I gave her a hug.
16
A New World
THAT NIGHT, CALLIE CURLED UP in her usual spot on the bed. Exhausted from the day of scanning, she immediately fell into a deep sleep, snoring softly. But I was still too jacked up; for the second night in a row, I didn’t sleep. Images of Callie’s brain danced in my head. But I had no idea what we had actually done. Dog brains were going to be our new world, but we had no map.
It turned out that the dogs’ brains looked nothing like a human’s brain. Apart from the size difference, many of the landmarks that I had become accustomed to seeing in human brains were either absent in the dogs or distorted into unrecognizable shapes. Now that we had dog brain scans, Andrew and I would have to grapple with interpreting all that data.
Dog brains and human brains differ in two important ways: structure and function. Brain structure refers to its shape. You don’t need to be a neuroscientist to see that humans and dogs have brains of different shapes. The brain structure consists of the different parts of the brain and their location relative to one another. This is why neuroscientists refer to prominent parts of the brain as landmarks. Obvious landmarks in all brains include the brainstem and spinal cord, the cerebellum, the ventricles (which make the cerebrospinal fluid), the corpus callosum (which connects the left and right sides of the brain), and a few structures in the basal ganglia—part of MacLean’s reptilian brain.
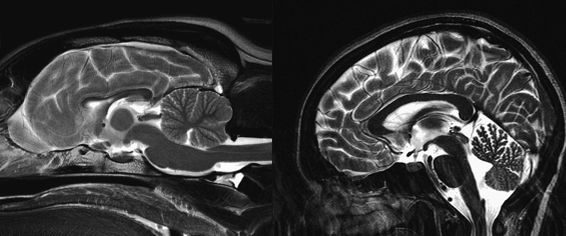
The dog brain (left) and the human brain (right). (Not to scale.)
(Dog brain image by permission of Thomas Fletcher, University of Minnesota; human brain by Gregory Berns)
But even with these landmarks, the largest part of the brain—the cerebral cortex—is radically different in dogs and humans. Presumably, that is what makes us different from each other.
Imagine comparing a map of the United States to a map of France. What can you deduce about these countries from looking at their maps? There is an obvious difference in size, but that doesn’t say much. Based on the arrangement of roads, the maps would give you a sense of where hubs of activity lie. Many roads lead to Paris, and you might correctly conclude that Paris is a key center in France. You would also notice important port cities like Marseille and Bordeaux and guess that these cities are centers of trade. In comparison, there is no obvious center of activity in the United States, but the road map would give you much of the same kind of information. The Northeast Corridor, from Washington, DC, to New York, immediately stands out, and you would be correct in assuming that this region is a critical center of government and economic activity. Similarly, coastal cities like Boston, Houston, Los Angeles, and San Francisco would stand out as centers of trade.
And so it is with the brains of dogs and humans. Even though we don’t know the exact function of different parts of the canine brain, we can make educated guesses based on what we have learned about other brains. Using landmarks that are common to both brains, we can begin to construct a more precise functional map of the dog brain.
But where to begin?
At first glance, dog brains didn’t look much like human brains at all, so it wasn’t apparent how much of the vast human neuroscience literature we could use. As I lay awake, I pictured the basic divisions of the human brain and tried to imagine how these might look in a dog’s brain. It was very much like looking at a map of a foreign country.
If we think of the brain as a gigantic computer, information goes in, the brain does something with it, and an action is produced, often in the form of movement. In this manner, inputs and outputs form the first great divide in the brain.
Inputs are relatively easy to understand. All information that flows into our brains must come through the five senses: vision, hearing, touch, smell, and taste. From the scientist’s point of view, inputs can be controlled during an experiment. For the experiment we had just accomplished, we controlled the visual channel by the hand signals we gave, and we controlled smell and taste by giving either peas or hot dogs.
Outputs are also easy to understand, especially if we consider movement as the main output of the brain. The earliest fMRI experiments had human subjects lying in the MRI and tapping their fingers for periods of thirty seconds. When the subjects tapped their fingers, activity in the part of the brain that controlled the hand was plainly visible.
The central sulcus is a groove in the human brain that runs almost vertically down the outside of each hemisphere. Everything behind the central sulcus is broadly concerned with inputs and everything in front with outputs. It is a defining landmark that divides the frontal lobe in front of the groove from the parietal lobe behind. The frontal bank of the central sulcus, it’s important to note, contains the neurons that control movement of all the parts of the body. Toward the bottom of this groove, above the ear, we find neurons that control the hand and mouth, and as we move up toward the crown of the head, we find neurons that control the legs. The neurons found along the sulcus control the opposite side of the body. When you move your right hand, a portion of the left central sulcus will become active, and this can be seen easily with fMRI.